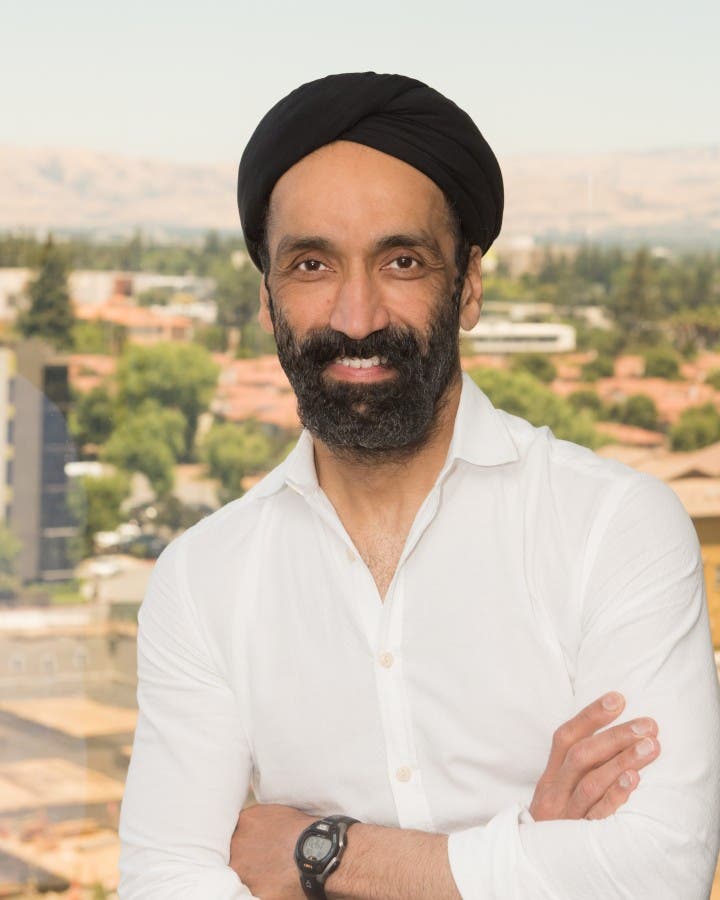
The share prices of EV start-ups have been rising sharply over recent months. Testa Inc., which produces 500,000 EVs per year is valued at more than US$800 billion. At one point, an EV start-up, Nikola, was worth US$40 billion, more than the market value of the 100-year old Ford Motor.
Investors have also taken notice of companies that develop batteries for these EVs. Start-ups that develop solid-state battery are attracting investors and existing car manufacturers as well as venture capital and SPACs.
A company that is developing solid-state battery is QuantumSpace Corporation Inc, which was a spin-off from Stanford University. The company is worth US$20.14 billion. The 10-K report, which the company submits to the SEC of the US, describes the state of the solid state battery and the major challenges that are facing companies to commercialize this new technology. The following is an extract of the 10-K report.
Corporate History and Background
On November 25, 2020, Kensington Capital Acquisition Corp., a SPAC merged with QuantumScape Battery, Inc. Later, Kensington Capital Acquisition Corp. changed its name to QuantumScape Corporation.
Overview
QuantumScape is developing next generation battery technology for electric vehicles (“EVs”) and other applications. We are at the beginning of a forecasted once-in-a-century shift in automotive powertrains, from internal combustion engines to clean EVs. While current battery technology has demonstrated the benefits of EVs, principally in the premium passenger car market, there are fundamental limitations inhibiting widespread adoption of battery technology. As a result, today, approximately 3% of global light-vehicles are electrified. We believe a new battery technology represents the most promising path to enable a mass market shift.
After 30 years of gradual improvements in conventional lithium-ion batteries we believe the market needs a step change in battery technology to make mass market EVs competitive with the fossil fuel alternative.
We have spent the last decade developing a proprietary solid-state battery technology to meet this challenge. We believe that our technology enables a new category of battery that meets the requirements for broader market adoption. The lithium-metal solid-state battery technology that we are developing is being designed to offer greater energy density, longer life, faster charging, and greater safety when compared to today’s conventional lithium-ion batteries.
Over the last eight years we have developed a strong partnership with Volkswagen Group of America Investments, LLC (“VGA”) and certain of its affiliates (together with VGA, “Volkswagen”). Volkswagen is one of the largest car companies in the world and intends to be a leader in EVs. Volkswagen has announced plans to launch more than 70 new EV models and build more than 25 million vehicles on electric platforms by the end of the decade. Over the last eight years Volkswagen has invested and committed to invest, subject, in certain cases, to certain closing conditions that have not yet been satisfied, a total of more than US$300 million in us and has established a 50-50 joint venture with us to enable an industrial level of production of our solid-state batteries. As 50-50 partners in the joint venture with Volkswagen, we expect to share equally in the revenue and profit from the joint venture. Over the course of our relationship, Volkswagen has successfully tested multiple generations of certain of our single-layer, laboratory cells at industry-accepted automotive rates of power (power is the rate at which a battery can be charged and discharged). We believe no other lithium-metal battery technology has demonstrated the capability of achieving automotive rates of power with acceptable battery life.
While we expect Volkswagen will be the first to commercialize vehicles using our battery technology, over the next few years as we build our initial pre-pilot manufacturing facility and our 1GWh pilot facility (the “Pilot Facility”), we intend to work closely with other automotive original equipment manufacturers (“OEMs”) to make our solid-state battery cells widely available over time. As part of our joint venture agreement we have agreed that the Pilot Facility will be the first commercial-scale facility to manufacture our battery technology for automotive applications, but, subject to the other terms of the joint venture arrangements, we are not limited from working in parallel with other automotive OEMs, or other non-automotive companies, to commercialize our technology. We recently have announced our plans to expand our manufacturing capability with the addition of a pre-pilot line facility in San Jose, CA (“QS-0”). QS-0 is intended to have a continuous flow, high automation line capable of building over 100,000 engineering cell samples per year. We expect to secure a long-term lease for QS-0 in the second half of this year and for QS-0 to be producing cells by 2023.
Our development uses earth-abundant materials and processes suitable for high volume production. Our processes use tools which are already used at scale in the battery or ceramics industries. Outside of the separator, our battery is being designed to use many of the materials and processes that are standard across today’s battery manufacturers. As a result, we expect to benefit from the projected industry-wide cost declines for these materials that result from process improvements and economies of scale. We believe that the manufacturing of our solid-state battery cells provides us with a structural cost advantage because our battery cells are manufactured without an anode.
Industry Background
Shift to EVs
We believe that evolving consumer preferences coupled with growing government incentives and regulations are driving a once-in-a-century shift to EVs.
Countries around the world are promoting EVs. The dependence on gasoline-powered internal combustion engine (“ICE”) vehicles has heightened environmental concerns, created reliance among industrialized and developing nations on large oil imports, and exposed consumers to unstable fuel prices and health concerns related to heightened emissions. Many national and regional regulatory bodies have adopted legislation to incentivize or require a shift to lower-emission and zero-emission vehicles. For example, countries such as the United Kingdom, the Netherlands, Sweden, Germany, and France have announced intentions to either increase applicable environmental targets or outright ban the sale of new ICE vehicles in the next two decades. More recently, California passed regulations requiring half of trucks sold in the state to be zero-emissions by 2035 and 100% by 2045.
This global push to transition from ICE vehicles, aided by favourable government incentives and regulations, is accelerating the growth in lower- and zero-emission vehicle markets.
Furthermore, consumers are increasingly considering EVs for a variety of reasons including better performance, growing EV charging infrastructure, significantly lighter environmental impact, and lower maintenance and operating costs. Automakers such as Tesla, Inc. have demonstrated that premium EVs can deliver a compelling alternative to fossil fuels. As EVs become more competitive and more affordable, we believe that they will continue to take market share from ICE vehicles. We believe that this shift will occur across vehicle types and market segments. However, some of the inherent limitations of lithium-ion battery technology remain an impediment to meaningful improvements in EV competitiveness and cost.
Current Battery Technology Will Not Meet the Requirements for Broad Adoption of EVs
Despite the significant progress in the shift to EVs, the market remains dominated by ICE vehicles. According to the International Energy Association, approximately 3% of light vehicles are EVs. For EVs to be adopted at scale across market segments batteries need to improve. In particular, we believe there are five key requirements to drive broad adoption of EVs:
• Battery capacity (energy density). EVs need to be able to drive over 300 miles on a single charge to achieve broad market adoption. The volume required for conventional lithium-ion battery technology limits the range of many EVs. Higher energy density will enable automotive OEMs to increase battery pack energy without increasing the size and weight of the vehicle’s battery pack.
• Fast charging capability. EV batteries need to be fast-charging to replicate the speed and ease with which a gasoline car can be re-fueled. We believe this objective is achieved with the ability to charge to at least 80% capacity in under 15 minutes, without materially degrading battery life.
• Safety (nonflammable). EV batteries need to replace as many of the flammable components in the battery as possible with non-flammable equivalents to reduce the extent of damage caused by a fire. With current batteries, many abuse conditions, including malfunctions that can result in overcharges and battery damage from accidents, can result in fires.
• Cost. Mass market adoption of EVs requires a battery that is capable of delivering long range while remaining cost competitive with a vehicle price point of around US$30,000.
• Battery life. Batteries need to be usable for the life of the vehicle, typically 12 years or 150,000 miles. If the battery fades prematurely, EVs will not be an economically practical alternative.
Since these requirements have complex interlinkages, most manufacturers of conventional lithium-ion batteries used in today’s cars are forced to make tradeoffs. For example, conventional batteries can be fast-charged, but at the cost of significantly limiting their battery life.
We believe that a battery technology that can meet these requirements will enable an EV solution that is much more broadly competitive with internal combustion engines. With more than 90 million ICE vehicles produced in 2019 across the auto industry, there is significant untapped demand for a battery that meets these goals – a potential market opportunity in excess of US$450 billion annually.
Limitations of Conventional Lithium-ion Battery Technologies
The last significant development in battery technology was the commercialization of lithium-ion batteries in the early 1990s which created a new class of batteries with higher energy density. Lithium-ion batteries have enabled a new generation of mobile electronics, efficient renewable energy storage, and the start of the transition to electrified mobility.
Since the 1990s, conventional lithium-ion batteries have seen a gradual improvement in energy density. Most increases in cell energy density have come from improved cell design and incremental improvements in cathode and anode technology.
However, there is no Moore’s law in batteries – it has taken conventional lithium-ion batteries at least 10 years to double in energy density and it has been approximately 30 years since the introduction of a major new chemistry. As the industry approaches the theoretical limit of achievable energy density for lithium-ion batteries involving carbon, we believe a new architecture is required to deliver meaningful gains in energy density.
Batteries have a cathode (the positive electrode), an anode (the negative electrode), a separator which prevents contact between the anode and cathode, and an electrolyte which transports ions but not electrons. A conventional lithium-ion battery uses a liquid electrolyte, a polymer separator, and an anode made principally of carbon (graphite) or a carbon/silicon composite. Lithium ions move from the cathode to the anode when the battery is charged and vice versa during discharge.
Conventional Lithium-Ion Battery Design
The energy density of conventional lithium-ion batteries is fundamentally limited by the anode, which provides a host material to hold the lithium ions, preventing them from binding together into pure metallic lithium. Metallic lithium, when used with conventional liquid electrolytes and porous separators, can form needlelike crystals of lithium known as dendrites, which can penetrate through the separator and short-circuit the cell.
While using a host material is an effective way to prevent dendrites, this host material adds volume and mass to the cell, it adds cost to the battery, and it limits the battery life due to side reactions at the interface with the liquid electrolyte. The rate at which lithium diffuses through the anode also limits the maximum cell power.
The addition of silicon to a carbon anode provides a modest boost to energy density relative to a pure carbon anode. However, silicon is also a host material that not only suffers from the limitations of carbon as discussed above, but also introduces cycle life challenges as a result of the repeated expansion and contraction of the silicon particles, since silicon undergoes significantly more expansion than carbon when hosting lithium ions. Furthermore, the voltage of the lithium-silicon reaction subtracts from the overall cell voltage, reducing cell energy.
Lithium-Metal Anode Required to Unlock Highest Energy Density
We believe that a lithium-metal anode is the most promising approach that can break out of the current constraints inherent in conventional lithium-ion batteries and enable significant improvements in energy density.
In a lithium-metal battery, the anode is made of metallic lithium; there is no host material. Eliminating the host material reduces the size and weight of the battery cell and eliminates the associated materials and manufacturing costs. This results in the highest theoretical gravimetric energy density for a lithium-based battery system. Lithium-ion batteries currently used in the auto industry have energy densities of less than 300 Wh/kg. We believe lithium-metal batteries have the potential to significantly increase this energy density.
Lithium-metal anodes are compatible with conventional cathode materials, and lithium-metal batteries will derive some benefit from continued improvement in conventional cathodes. Moreover, lithium-metal anodes may enable future generations of higher energy cathodes that cannot achieve energy density gains when used with lithium-ion anodes,.
Although the industry has understood for 40 years the potential benefits of lithium-metal anodes, the industry has not been able to develop a separator that makes a lithium-metal anode practical for automotive use.
Solid-State Separator Required to Enable Lithium-Metal Anode
We believe that a lithium-metal battery requires that the porous separators used in current lithium-ion batteries be replaced with a solid-state separator capable of conducting lithium ions between the cathode and anode at rates comparable to conventional liquid electrolyte while also suppressing the formation of lithium dendrites. While various solid-state separators have been shown to operate at low power densities, such low power densities are not useful for most practical applications. To our knowledge, we are the only company that has been able to demonstrate a solid-state separator for lithium-metal batteries that reliably prevents dendrite formation at higher power densities, such as those required for automotive applications and fast-charging.
We believe that our ability to develop this proprietary solid-state separator will enable the shift from lithium-ion to lithium-metal batteries.
Our Technology
Our proprietary solid-state lithium-metal cell represents the next-generation of battery technology.
Our battery cells have none of the host materials used in conventional anodes. In fact, when our cells are manufactured there is no anode; lithium is present only in the cathode. When the cell is first charged, lithium moves out of the cathode, diffuses through our solid-state separator and plates in a thin metallic layer directly on the anode current collector, forming an anode. When the battery cell is discharged, the lithium diffuses back into the cathode.
Eliminating the anode host material found in conventional lithium-ion cells substantially increases the volumetric energy density. A pure lithium-metal anode also enables the theoretically highest gravimetric energy density for a lithium battery system.
Our proprietary solid-state separator is the core technology breakthrough that enables reliable cycling of the lithium-metal anode battery. Without a working solid-state separator, the lithium would form dendrites which would grow through a traditional porous separator and short circuit the cell.
An effective solid-state separator requires a solid material that is as conductive as a liquid electrolyte, chemically stable next to lithium–one of the most reactive elements–and able to prevent the formation of dendrites. Our team worked over ten years to develop a composition that meets these requirements and to develop the techniques necessary to manufacture the separator material at scale using a continuous process. We have a number of patents covering both the composition of this material and key steps of the manufacturing process.
Our solid-state separator is a dense, entirely inorganic ceramic. It is made into a film that is thinner than a human hair and then cut into pieces about the length and width of a playing card. Our solid-state separator is flexible because it has a low defect density and is thin. In contrast, typical household ceramics are brittle and can break due to millions of microscopic defects which reduce structural integrity.
The separator is placed between a cathode and anode current collector to form a single battery cell layer. These single layers will be stacked together into a multilayer cell, about the size of a deck of cards, that will be the commercial form factor for EV batteries.
Our cathodes use a combination of conventional cathode active materials (NMC) with an organic gel made of an organic polymer and organic liquid catholyte. In the future, we may use other compositions of cathode active materials, including cobalt-free compositions. We have an ongoing research and development investigation into inorganic catholyte that could replace the organic gel made of an organic polymer and organic liquid catholyte currently used.
As communicated in our solid-state battery showcase event on December 8, 2020, our single-layer solid-state cells have been extensively tested for power density, cycle life, and temperature performance. This is the only solid-state cell we are aware of that has been validated to run at automotive power densities by a leading automotive OEM. In addition, we believe our battery technology may provide significant improvements in energy density compared to today’s conventional lithium-ion batteries.
Benefits of Our Technology
We believe our battery technology will enable significant benefits across battery capacity, life, safety, and fast charging while minimizing cost. We believe these benefits will provide significant value to automotive OEMs by enabling greater customer adoption of their EVs. By solving key pain-points such as 15-minute fast charging, we believe our battery echnology will enable the delivery of an EV experience that is significantly more competitive with fossil fuel vehicles than what today’s EVs can achieve with conventional batteries.
Our battery technology is intended to meet the five key requirements we believe will enable mass market adoption of EVs:
• Energy density. Our battery design is intended to significantly increase volumetric and gravimetric energy density by eliminating the carbon/silicon anode host material found in conventional lithium-ion cells. This increased energy density will enable EV manufacturers to increase range without increasing the size and weight of the battery pack, or to reduce the size and weight of the battery pack which will reduce the cost of the battery pack and
other parts of the vehicle. For example, we estimate that our solid-state battery cells will enable a car maker to increase the range of a luxury performance EV—with 350 litres of available battery space—from 250 miles (400 km) to 450 miles (730 km) without increasing the size and weight of the battery pack. In the same example, our battery would enable the car maker to increase the maximum power output of such a vehicle from 420 kW to 650 kW without increasing the size of the battery pack. Alternatively, we believe that our solid-state battery cells will enable a car maker to increase the range of a mass market sedan—with 160 litres of available battery space—from 123 miles (200km) to 233 miles (375km) without increasing the size and weight of the battery pack. Similarly, our battery would enable the car maker to increase the maximum power output of such vehicle from 100 kW to 150 kW without increasing the size of the battery pack.
• Battery life. Our technology is expected to enable increased battery life relative to conventional lithium-ion batteries. In a conventional cell, battery life is limited by the gradual irreversible loss of lithium due to side reactions between the liquid electrolyte and the anode. By eliminating the anode host material, we expect to eliminate the side reaction and enable longer battery life. Our latest single-layer prototype cells have been tested to over 1000
cycles (under stringent test conditions, including 100% depth-of-discharge cycles at one-hour charge and discharge rates at 30 degrees Celsius with commercial-loading cathodes) while still retaining over 80% of the cells’ discharge capacity. This performance exceeds the cycle life and capacity retention in many EV battery warranties today, which may be to 150k miles to 70% of the cells’ discharge capacity.
• Fast charging capability. Our battery technology, and specifically our solid-state separator material, has been tested to demonstrate the ability to charge to approximately 80% in 15 minutes, significantly faster than commonly used high-energy EV batteries on the market. In these conventional EV batteries, the limiting factor for charge rate is the rate of diffusion of lithium ions into the anode. If a conventional battery is charged beyond these limits, lithium can start plating on carbon particles of the anode rather than diffuse into the carbon particles. This causes a reaction between the plated lithium and liquid electrolyte which reduces cell capacity and increases the risk of dendrites that can short circuit the cell. With a lithium-metal anode, using our solid-state separator, we expect the lithium can be plated as fast as the cathode can deliver it.
• Increased safety. Our solid-state battery cell uses a ceramic separator which is not combustible and is therefore safer than conventional polymer separators. This ceramic separator is also capable of withstanding temperatures considerably higher than those that would melt conventional polymer separators, providing an additional measure of safety. In high temperature tests of our solid-state separator material with lithium, the separator material remained stable in direct contact with molten lithium without releasing heat externally, even when heated up to 250 degrees, higher than the 180-degree melting point of lithium.
• Cost. Our battery technology eliminates the anode host material and the associated manufacturing costs, providing a structural cost advantage compared to traditional lithium-ion batteries. When comparing manufacturing facilities of similar scale, we estimate that eliminating these costs will provide a savings of approximately 17% compared to the costs of building traditional lithium-ion batteries at leading manufacturers.
Our Competitive Strengths
Only proven lithium-metal battery technology for automotive applications to our knowledge. We have built and tested over one hundred thousand single-layer solid-state cells and have demonstrated that our technology meets automotive requirements for power, cycle life, and temperature range. In 2018, Volkswagen announced it had successfully tested certain of our single-layer, laboratory battery cells at automotive rates of power.
Partnership with one of the world’s largest automotive OEMs. We are partnered with Volkswagen, one of the largest automakers in the world. Volkswagen has been a collaboration partner and major investor since 2012 and has invested or committed to invest, subject, in certain cases, to certain closing conditions that have not yet been satisfied, a total of more than $300 million. In addition, Volkswagen has committed additional capital to fund our joint venture. Volkswagen plans to launch more than 70 new electric models and build more than 25 million vehicles on electric platforms by the end of the decade. Together with Volkswagen, we have established a joint venture to enable an industrial level of production of our solid-state batteries for use in Volkswagen vehicles. As 50-50 partners in the joint venture with Volkswagen, we expect to share equally in the revenue and profit from the joint venture.
High barriers to entry with extensive patent and intellectual property portfolio. Over the course of 10 years, we have generated more than 200 U.S. and foreign patents and patent applications – including broad fundamental patents around our core technology. Our proprietary solid-state separator uses the only material we know of that can cycle lithium at automotive current densities without forming dendrites. Our battery technology is protected by a range of patents, including patents that cover:
• Composition of matter, including the optimal composition as well as wide-ranging coverage of a number of variations;
• Enabling battery technology covering compositions and methods required to incorporate a solid-state separator into a battery;
• Manufacturing technology, protecting the way to make the separator at scale using roll-to-roll processes, without semiconductor style production or batch processes used in traditional ceramics; and
• Material dimensions, including our proprietary solid-state separator, covering any separator with commercially practical thicknesses for a solid-state battery.
Significant development focused on next-gen technology for automotive applications. We have spent over ten years and over $300 million developing our battery technology. We have run over 2.6 million tests on over 700,000 cells and cell components. Our technical team comprises more than 250 employees, many of whom have worked at large battery manufacturers and automotive OEMs. Through its experience, our team has significant technical know-how and is supported by extensive facilities and equipment, development infrastructure, and data analytics.
Designed for volume production. Our technology is designed to use earth-abundant materials and processes suitable for high volume production. Our processes use tools which are already used at scale in the battery or ceramics industries. While preparing for scale production, we have purchased or tested production-intent tools from the world’s leading vendors. In particular, we expect to produce our proprietary separator using scalable continuous processing. Although our separator material is proprietary, the inputs are readily available and can be sourced from multiple suppliers across geographies.
Structural cost advantage leveraging industry cost trends. Aside from the separator, our battery is being designed to use many of the materials and processes that are standard across today’s battery manufacturers. As a result, we expect to benefit from the projected industry-wide cost declines for these materials that result from process improvements and economies of scale. We believe that the manufacturing of our solid-state battery cells provides us with a structural cost advantage because our battery cells are manufactured without an anode.
Our Growth Strategy
Continue to develop our commercial battery technology. We will continue developing our battery technology with the goal of enabling commercial production in 2024. We have validated capabilities of our solid-state separator and battery technology in single-layer solid-state cells at the commercially required size (70x85mm) and four-layer solid-state battery cells at a smaller size (30x30mm). We must now develop multi-layer cells with commercial dimensions and many more layers, to continue improving yield and performance and to optimize all components of the cell for high volume manufacturing. We will continue to work to further develop and validate the volume manufacturing processes to enable high volume manufacturing and minimize manufacturing costs. We will continue to work on increasing the yield of our separators to reduce scrappage and to increase utilization of manufacturing tools. Our current funds will enable us to expand and accelerate research and development activities and undertake additional initiatives. Finally, we will continue to use our engineering line in San Jose, California to prepare for high volume manufacturing and plan our first commercial production Pilot Facility through our joint venture partnership with Volkswagen. In addition, we expect that our recently announced QS-0 facility will help provide the additional capacity we need for our development work and will enable us to accelerate work on the next-generation of manufacturing tools. QS-0 is also intended to provide capacity to make enough batteries for hundreds of long-range battery electric test vehicles per year. This will allow us to provide early cells to Volkswagen, as well as other automotive partners, explore non-automotive applications, and help de-risk subsequent commercial scale-up. We expect to secure a long-term lease for QS-0 in the second half of this year and for QS-0 to be producing cells by 2023.
Meet Volkswagen battery demand. The Pilot Facility to be built and run by QSV Operations LLC (“QSV”) and the subsequent 20GWh expansion of the Pilot Facility (the “20GWh Expansion Facility”) would represent a small fraction of Volkswagen’s demand for batteries and implies vehicle volumes under 2% of Volkswagen’s total production in 2019, assuming a 100KWh pack size. Our goal is to significantly expand the production capacity of the joint venture, in partnership with Volkswagen, to meet more of their projected demand.
Expand partnerships with other automotive OEMs. While we expect Volkswagen will be the first to commercialize vehicles using our battery technology, over the next few years as we build our Pilot Facility, we intend to work closely with other automotive OEMs to make our solid-state battery cells widely available over time. As part of our joint venture agreement we have agreed that the Pilot Facility will be the first commercial-scale facility to manufacture our battery technology for automotive applications, but, subject to the other terms of the joint venture arrangements, we are not limited from working in parallel with other automotive OEMs to commercialize our technology. We expect that QS-0 will allow us to provide early cells to Volkswagen, as well as other automotive partners, explore non-automotive applications, and help de-risk subsequent commercial scale-up.
Expand target markets. We are currently focused on automotive EV applications, which have the most stringent set of requirements for batteries. However, we recognize that our solid-state battery technology has applicability in other large and growing markets including stationary storage and consumer electronics such as smartphones and wearables.
Expand commercialization models. Our technology is being designed to enable a variety of business models. In addition to joint ventures, such as the one with Volkswagen, we may operate solely-owned manufacturing facilities or license technology to other manufacturers, such as our recently announced QS-0 facility that is planned for the San Jose area. Where appropriate, we may build and sell separators rather than complete battery cells.
Continued investment in next-gen battery innovation. We intend to continue to invest in research and development to improve battery cell performance, improve manufacturing processes, and reduce cost.
Manufacturing and Supply
Our battery manufacturing process is being designed to be very similar to that of conventional lithium-ion battery manufacturing, with a few exceptions:
• We use a proprietary separator material instead of the polypropylene separator used in lithium-ion cells.
• Our architecture eliminates the need for anode manufacturing, reducing capital investment and lowering operating costs.
• We will build our multi-layer cells by sequentially stacking separators, cathodes and current collectors rather than winding these materials together.
• Our cell design allows us to greatly shorten the weeks-long aging process required for conventional lithium-ion cells, thus decreasing manufacturing cycle time and reducing working capital needs.
Our architecture depends on our proprietary separator, which we will manufacture ourselves. Though our separator design is unique, its manufacturing relies on well-established, high-volume production processes currently deployed globally in other industries.
We plan to source our input materials from industry leading suppliers to the lithium-ion battery industry, and we already have strategic relationships in place with the industry’s leading vendors of cathode material, the most critical purchased input to our cell, along with leading vendors of other less critical inputs. Our separator is made from abundant materials produced at industrial scale in multiple geographies. We do not anticipate any unique supply constraints that would impede the commercialization of our product for the foreseeable future.
Research and Development
We conduct research and development at our headquarters facility in San Jose, California. Research and development activities concentrate on making further improvements to our battery technology, including improvements to battery performance and cost.
Our research and development currently includes programs for the following areas:
• Multi-layering. To date, we have only produced single-layer solid-state cells at the commercially required size (70x85mm) and four-layer solid-state cells at a smaller size (30x30mm). In order to produce commercially-viable solid-state battery cells for automotive applications, we must produce multilayer battery cells which may range from several dozen to over one hundred layers, depending on our customers’ requirements, and to do so in the commercially required size. We will need substantial development and to overcome the challenges in creating these cells and implement the appropriate cell design for our solid-state battery cell.
• Improved yields. We are focused on improving the yields (useful output) of both our solid-state separators and our battery cells. We are automating our manufacturing process and purchasing larger-scale manufacturing equipment. We will need to significantly increase our yield before we can manufacture our solid-state battery cells in volume.
• Continued improvement in the solid-state separator. We are working to improve the reliability and performance of our solid-state separator, including decreasing the thickness. We have selected a method of continuous processing found at scale in both the battery and ceramic industries and are working on continuous improvement of this process. In addition, we are investigating alternative processing methods that may further increase the capital efficiency of the process.
• Continued improvement of the cathode. Our cathodes use a combination of conventional cathode active materials (NMC) along with an organic gel made of an organic polymer and organic liquid catholyte. In the future, we may use other cathode active materials, including cobalt-free compositions. We have an ongoing research and development investigation into inorganic catholyte that could replace the organic gel made of an organic polymer and organic liquid currently used.
• Integration of advanced cathode materials. We plan to benefit from industry cathode chemistry improvements and/or cost reduction. Our solid-state separator platform is being designed to enable some of the most promising next-generation cathode technologies, including high voltage or high capacity cathode active materials, which when combined with a lithium-metal anode, may further increase cell energy densities.
Intellectual Property
The success of our business and technology leadership is supported by our proprietary battery technology. We rely upon a combination of patent, trademark and trade secret laws in the United States and other jurisdictions, as well as license agreements and other contractual protections, to establish, maintain and enforce rights in our proprietary technologies. In addition, we seek to protect our intellectual property rights through nondisclosure and invention assignment agreements with our employees and consultants and through non-disclosure agreements with business partners and other third parties. We regularly file applications for patents and have a significant number of patents in the United States and other countries where we expect to do business. Our patent portfolio is deepest in the area of solid-state separators with additional areas of strength in anodes, next-generation cathode materials, and cell, module, and pack design specific to lithium-metal batteries. Our trade secrets primarily cover manufacturing methods.
As of December 31, 2020, we owned or licensed, on an exclusive basis, 80 issued U.S. patents and 40 pending or allowed U.S. patent applications, and 103 granted foreign patents and patent applications. We have 1 registered U.S. trademark and 6 pending U.S. trademark applications. Our issued patents start expiring in 2033.
Competition
The EV market, and the battery segment in particular, is evolving and highly competitive. With the introduction of new technologies and the potential entry of new competitors into the market, we expect competition to increase in the future, which could harm our business, results of operations, or financial condition.
Our prospective competitors include major manufacturers currently supplying the industry, automotive OEMs and potential new entrants to the industry. Major companies now supplying batteries for the EV industry include Panasonic Corporation, Samsung SDI, Contemporary Amperex Technology Co. Limited, and LGChem Ltd. They supply conventional lithium-ion batteries and in many cases are seeking to develop solid-state batteries, including potentially lithium-metal batteries. In addition, because of the importance of electrification, most automotive OEMs are researching and investing in solid-state battery efforts and, in some cases, in battery development and production. For example, Tesla, Inc. is building multiple battery gigafactories and potentially could supply batteries to other automotive OEMs, and Toyota Motors and a Japanese consortium have a multi-year initiative pursuing solid-state batteries.
A number of development-stage companies are also seeking to improve conventional lithium-ion batteries or to develop new technologies for solid-state batteries, including lithium-metal batteries. Potential new entrants are seeking to develop new technologies for cathodes, anodes, electrolytes and additives. Some of these companies have established relationships with automotive OEMs and are in varying stages of development.
We believe our ability to compete successfully with lithium-ion battery manufacturers and with other companies seeking to develop solid-state batteries will depend on a number of factors including battery price, safety, energy density, charge rate and cycle life, and on non-technical factors such as brand, established customer relationships and financial and manufacturing resources.
Many of the incumbents have, and future entrants may have, greater resources than we have and may also be able to devote greater resources to the development of their current and future technologies. They may also have greater access to larger potential customer bases and have and may continue to establish cooperative or strategic relationships amongst themselves or with third parties (including automotive OEMs) that may further enhance their resources and offerings.
Government Regulation and Compliance
There are government regulations pertaining to battery safety, transportation of batteries, use of batteries in cars, factory safety, and disposal of hazardous materials. We will ultimately have to comply with these regulations to sell our batteries into the market. The license and sale of our batteries abroad is likely to be subject to export controls in the future.