McKinsey: Women more likely to be replaced by AI than men
Posted on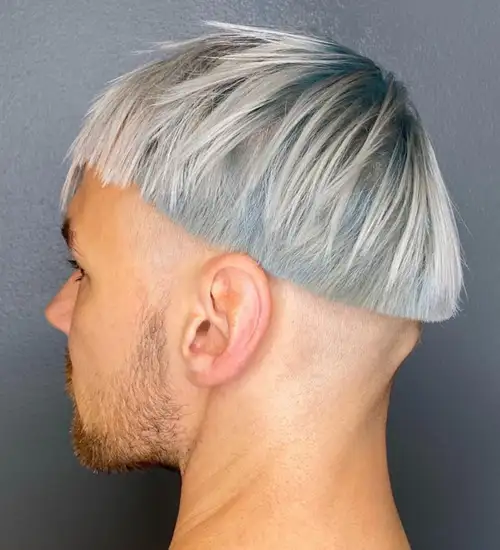
12 million jobs to be automated in the US alone over next seven years, McKinsey says
Women are 50pc more likely than men to lose their jobs in the artificial intelligence (AI) race, according to a new study that predicts millions more roles will be automated by 2030.
McKinsey said around 12 million jobs will be replaced by AI in the US alone over the next seven years.
The management consultancy said women will be more affected by companies replacing staff with chatbots because they are more likely to hold “lower-wage jobs”.
Other jobs heavily represented by women, including customer service roles and secretaries are also in the firing line, according to McKinsey.
McKinsey’s study, published this week, said women are “heavily represented” in those two sectors, with potentially 5.7m jobs being lost in those areas alone by 2030.
McKinsey said: “Workers in lower-wage jobs are up to 14 times more likely to need to change occupations than those in highest-wage positions, and most will need additional skills to do so successfully.”
Women are 1.5 times more likely overall to be forced to change jobs as a result of AI-powered automation changing how companies recruit and use human workers.
Those without university degrees, as well as the youngest and oldest workers are also at higher risk of losing their jobs to technology such as AI-powered chatbots.
Others in professional roles such as management, healthcare and the legal profession are least likely to be impacted, McKinsey said.
Not all professional roles are immune. Figures from jobs board Adzuna suggest that graphic designers, software engineers and advertising specialists are at greatest risk from AI.
Traditionally, technological advancements result in jobs being created in other sectors instead of being destroyed altogether.
Public availability of AI tools such as ChatGPT have accelerated the trend for job automation, with McKinsey revising its job loss predictions upwards by a quarter from estimates it made in 2021.
My MBA students also have pointed out that their companies are also applying AI to replace humans in many activities. One example is the automation of travel plans. However, their bosses still have the luxuries of secretaries and personal assistants to plan their travels!!!
Some jobs will be safe like a barber, unless you want to have the hairstyles of Lauren and Hardy, a mushroom cut.